Colloid physics
Explore the dynamics of soft matter and colloid properties of emulsions, gels, biomaterials and liquid crystals with SENSOCELL optical tweezers.
Measure colloidal particle interactions and the rheological properties of colloids
Optical tweezers can measure the force-displacement relationship of a colloidal particle in a fluid or gel, by applying a controlled displacement to the trapped particle and measuring the resultant force. By monitoring the particle’s response to these forces (e.g., its position fluctuations), researchers can deduce the viscoelastic G modulus (elastic and viscous components) of the surrounding medium.
Additionally, Sensocell optical tweezers allow for microrheological experiments by tracking the movement of colloidal particles and assessing how the medium resists or responds to deformation. This technique is particularly valuable in complex fluids like biological gels or polymers, where traditional rheology methods are difficult to apply.
Applications in Colloid Physics
- Understanding inter-particle interactions: Optical tweezers help study how colloidal particles interact with each other under various conditions (e.g., different temperatures, pH levels, or solvent environments).
- Probing phase transitions: Researchers can use optical tweezers to investigate how colloids transition between different states, such as from liquid to gel or crystal-like arrangements, and how these transitions affect the material’s properties.
- Studying soft matter systems: Optical tweezers are very useful for exploring the dynamics of soft matter, including colloids, emulsions, gels or liquid crystals which have applications in materials science, biology, and industry.
Selected publications:
- Hunter Seyforth, Mauricio Gomez, W. Benjamin Rogers, Jennifer L. Ross, and Wylie W. Ahmed. Nonequilibrium fluctuations and nonlinear response of an active bath. Phys. Rev. Research 4 (2022).
- Ophelie Squillace, Ting Zhang, Lia Argentou, Andrew Ward, Zhenyu J. Zhang. Nanoscopic wetting behaviour of single oil droplets on a fibre. Colloids and Surfaces A: Physicochemical and Engineering Aspects (2024).
Dynamics of an E. coli active colloid bath
In this example, Dr Ahmed (Université de Toulouse; Slam-Lab) et al., taken from the paper titled “Nonequilibrium fluctuations and nonlinear response of an active bath“ explore the dynamics of a passive colloidal probe immersed in an active bath composed of E. coli bacteria.
The authors use optical tweezers and light momentum analysis to study the interactions between the colloidal probe and the surrounding active bath, comparing these results with those obtained from a passive bath (water).
This research provided experimental evidence of how active matter, such as a suspension of E. coli, can induce complex, nonequilibrium dynamics in immersed particles. This has implications for understanding the behavior of systems far from equilibrium.
Nonequilibrium fluctuations in active colloid
The passive probe in the active bath exhibited enhanced nonequilibrium fluctuations compared to the passive bath. These fluctuations are driven by the activity of the bacteria and deviate significantly from what is expected in equilibrium systems. The authors measured the active energy spectrum for the quantification of the nonthermal energetic fluctuations of a colloid in the active bath
Nonlinear rheology
The viscosity of the active bath varied with the Péclet number (Pe), a dimensionless number representing the ratio of advective to diffusive transport rates. The study observed shear thinning at low Pe values, followed by shear thickening at intermediate Pe values, and a plateau in viscosity at high Pe values. This behavior contrasts with the passive bath, where no such variations were noted.
Force fluctuations
The distribution of force fluctuations in the active bath depended on Pe, with higher Pe leading to wider distributions, indicating more significant deviations from thermal equilibrium.
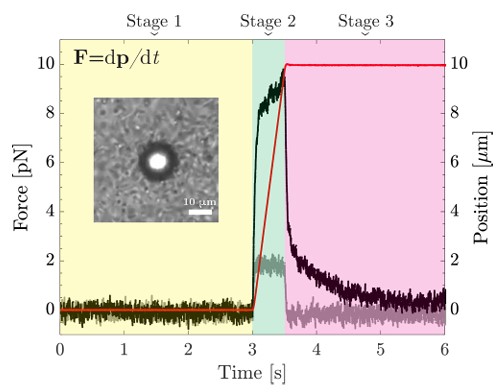
Fig. 1 Overview of the experimental protocol: spontaneous force fluctuations are recorded in stage 1 (yellow shaded), nonlinear mechanical response is assessed in stage 2 (green shaded), and force relaxation is analyzed in stage 3 (magenta shaded).
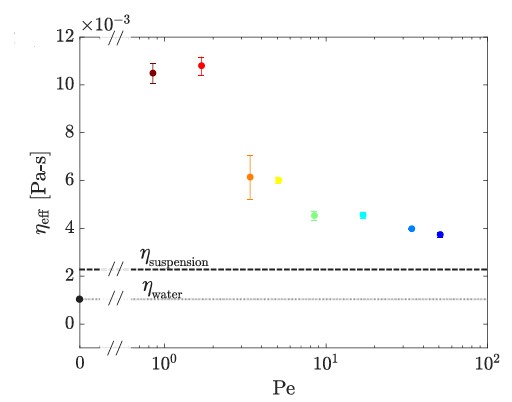
Fig. 2 Effective viscosity of an active colloid bath (E.coli) as a function of Pe number.
Interaction of an emulsion droplet colloid with fiber
This example taken from the paper titled “Nanoscopic wetting behaviour of single oil droplets on a fibre” published in Colloids and Surfaces A Journal. In this work, researchers from from the University of Birmingham and the Central Laser Facility at the Rutherford Appleton Laboratory studied the interactions between silicone oil droplets and microscopic fibers, focusing on the wetting and surface interactions under various conditions. Using optical tweezers combined with our direct force sensor based on momentum analysis, the researchers quantified these interactions.
The study examined how factors such as surfactant chemistry, pH, and salt concentration influence the behavior of silicone droplets when they come into contact with different fiber types, particularly cotton and polyester.
Oil droplet colloids-fiber interactions depend on electrostatic forces
The interaction between the oil droplets and fibers is largely influenced by the electrostatic forces, which can be modulated by changing the salt concentration in the surrounding medium. Indeed, higher salt concentrations reduced the electrostatic repulsion, leading to increased adhesion and spreading of droplets on the fibers. Different fibers exhibited varying degrees of interaction, with polyester showing stronger negative surface charge compared to cotton, affecting how the droplets interacted with each fiber type.
The study successfully demonstrated that optical tweezers could be used to directly measure and quantify these interactions at a microscopic scale, providing valuable insights into the wetting behavior of emulsion droplet colloids on fibers.
Images are courtesy of Andy Ward (CLF Lasers for Science Facility, UK).
Check the related video example:
Video. Interaction between a fibre and an oil droplet.
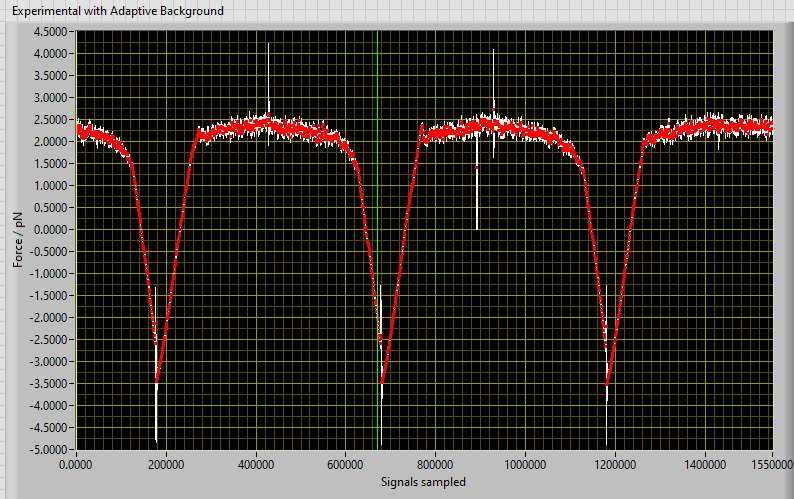
Fig.1 Cell-cell interaction between a cancer cell and a T-cell. The cell-cell adhesion force is given by the drop in the force signal (red line data) when the two cells are separated.
Rheology of water:glycerol mixtures and polyacrylamide gels colloids
In this experiment, 3-micron latex beads were added to different water:glycerol mixtures (0%, 20%, 40%, 60% and 80% glycerol in water) and used as probes to characterize the rheological properties of the gels. This work is in collaboration with BIOPT lab from the University of Barcelona.
For each single experiment, a bead was trapped using our SENSOCELL optical tweezers and forced to oscillate sinusoidally with 200 nm amplitude at increasing frequencies (3Hz to 100 Hz) launching our TimSom acive rheology built-in routine. The calculated viscosity values were then obtained by fitting the frequency dependence of the measured loss modulus (G”).
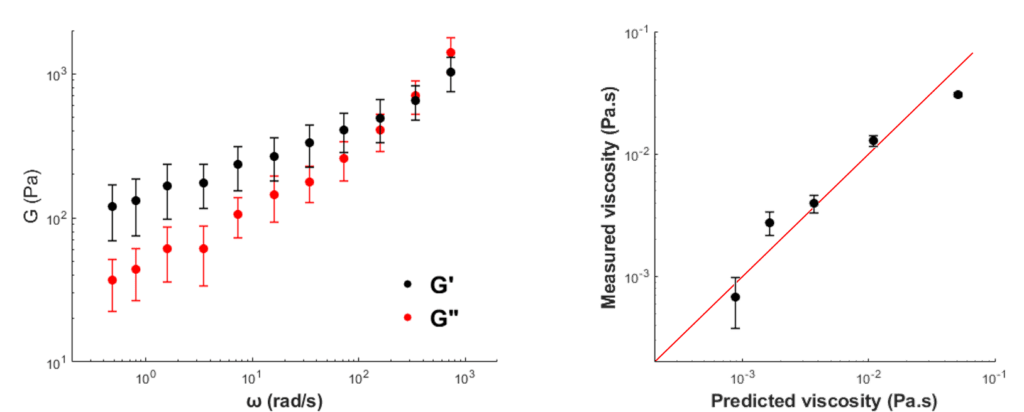
Fig. 1 Left: example of measured loss moduli at increasing frequencies for a 40% glycerol mixture. Right: predicted versus measured viscosity values of the different mixtures (increasing viscosity values correspond to increasing glycerol percentages). Symbols indicate mean of 9 different beads probed, error bars indicate S.D.
Viscoelastic properties of soft gels
In a similar fashion, we performed active micro-rheology experiments inside polyacrylamide gels with embedded micrometer beads to get their complex shear moduli.
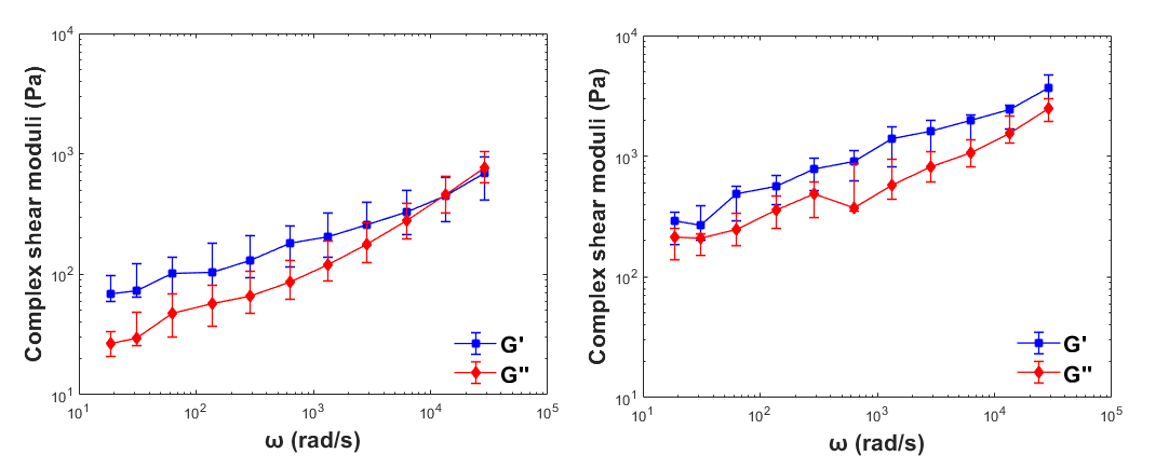
Fig 2. Example of the frequency-dependent behavior of the complex shear moduli of soft polyacrylamide gels. Blue symbols indicate storage modulus (G’) and red symbols indicate loss modulus (G”). Symbols are median values and error bars indicate Q1 and Q3 ranges. N = 13 beads probed for this experiment.
Rheology of a Liquid Crystal colloid
Coming soon. Contact us for more information.
Key Concepts
-
- Colloid: A mixture where tiny particles (1-1000 nm) are dispersed throughout a continuous medium, remaining evenly distributed without settling.
- Soft Matter: Materials, such as liquids, polymers, gels, and colloids, that deform easily under external forces due to weak intermolecular interactions.
- Microrheology: The study of material flow and deformation on a microscopic scale, often using the motion of probe particles to measure local viscoelastic properties.
- Active Bath: A system where passive particles are immersed in a medium containing active components (e.g., bacteria) that drive non-equilibrium dynamics through their motion.
- Gel: A soft, solid-like material consisting of a network of crosslinked polymers that trap a large amount of liquid, exhibiting both elastic and viscous properties.
Advantages
-
- Direct force measurements: SENSOCELL’s direct force sensor allows measuring forces on different samples without requiring calibration independently of the medium physical properties (like viscosity or refraction index).
- Precision: SENSOCELL offer precise control over the position and movement of individual cells, allowing for detailed manipulation and measurement of cell-cell interactions.
- Single particle analysis: laser tweezers can isolate and study individual particles, providing insights into heterogeneity within a sample that might be masked in bulk measurements.
- Sensitivity: optical tweezers can apply forces in the piconewton (pN) range, suitable for probing the weak interactions in soft matter systems.
- Variety of environments: Sensocell can be used in different media, including liquids, gels, liquid crystals and biological samples, making them versatile for various colloid systems.
- Multifunctional: SENSOCELL optical tweezers can be combined with other techniques, such as fluorescence microscopy.
- Nonlinear responses: the technique is well-suited to exploring nonlinear mechanical responses and other complex phenomena that arise in colloidal systems
Conclusions
Sensocell is a powerful tool in the field of colloid physics, particularly for studying the mechanical and dynamic properties of colloidal particles. Its ability to manipulate particles with high precision and minimal disturbance, combined with the potential for real-time and quantitative analysis, allows for a deeper understanding of colloidal systems.
Would you like a DEMO?
Download SENSOCELL brochure