Cell rigidity and elasticity
Perform automatized indentation and stretching experiments to measure cell rigidity and elasticity in an easy and straight forward manner.
Cell rigidity and elasticity measurements via indentation and stretching assays
Measuring cell rigidity and elasticity provides vital information about cellular behavior including disease mechanisms, cell migration, cell differentiation or signaling pathways.
Cell indentation
In cell indentation assays using optical tweezers, a small bead, usually made of polystyrene or silica, is trapped and positioned above the cell membrane. To facilitate its attachment to the cell membrane, the bead can be coated with a substance. Next, the optical tweezers push the bead into the cell membrane. The displacement of the bead controls the depth of indentation. As the bead indents the cell, the optical tweezers measure the exerted force. Both the applied force and the resulting indentation are recorded, and the data is plotted to create force-indentation curves. These curves offer insights into the mechanical properties of the cell. To determine the cell’s rigidity, one measures the force required to achieve a certain indentation. A stiffer cell requires more force to indent. Additionally, the relationship between the applied force and the resulting indentation is used to calculate the cell’s Young’s modulus, which measures its elasticity.
Cell stretching
Similarly, a cell stretching assay provides the same kind of information. This method requires two optical traps to stretch the cell. In traditional optical tweezer setups, two beads are attached to opposite sides of a cell. These beads are then trapped and controlled to move away from each other, stretching the cell membrane between them. However, with the integrated direct force sensor in SENSOCELL optical tweezers, cell stretching experiments can be conducted without beads, as the optical tweezers can directly trap the cell membrane from opposite sides and measure the forces exerted on it.
Selected publications:
- R. Ombid, G. Oyong, E. Cabrera, W. Espulgar, M. Saito, E. Tamiya, and R. Pobre, In-vitro study of monocytic THP-1 leukemia cell membrane elasticity with a single-cell microfluidic- assisted optical trapping system. Biomed. Opt. Express 11, 6027-6037 (2020).
Cell rigidity and elasticity measurements of leukemia cells
In this example, a custom optical tweezers setup designed by Impetux, combined with a microfluidic device, enabled precise and controlled force application on individual isolated leukemia cells. This allowed researchers to measure cellular stiffness and elasticity accurately. By trapping and indenting the cell membrane with micron-sized beads, they observed the deformation response and calculated the Young’s elastic modulus of the leukemia cells.
Young’s modulus of control and treated leukemia cells
This method helps understand the mechanical behavior of leukemia cells, which can differ significantly from healthy cells. These mechanical properties are crucial for understanding disease progression and developing therapeutic strategies targeting the mechanical aspects of cancer cells. In vitro cell membrane elasticity analysis indicated that THP-1 leukemia cells treated with Zeocin exhibited a lower elastic modulus compared to untreated cells. The reduction in membrane elasticity was attributed to ongoing cell apoptosis in THP-1 leukemia cells due to Zeocin. Read the full paper here.
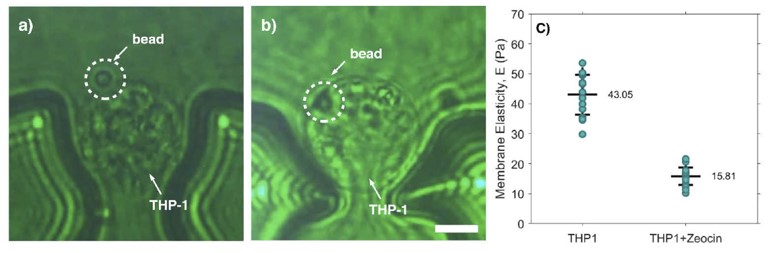
Video example of cell indentation assay and force-distance plot
The automated routines for controlling trap motion, including custom periodic oscillations and trajectories, along with the direct force spectroscopy technology integrated into the Sensocell optical tweezers, facilitate cell indentation assays with ease. In this video, we demonstrate the use of a 3µm polystyrene bead to indent a yeast cell. The bead is trapped and positioned a few microns away from the cell surface. Subsequently, a predefined back-and-forth motion is applied to the bead. All actions are managed through the camera interface. During the experiment, the force-distance plot on the left displays the data in real-time. Positive forces indicate that the bead is pushing against the cell, while negative forces indicate the bead is detaching from the cell surface. Several binding events are observed, shown by the force jumping back to zero after detachment.
Cell rigidity and cell elasticity quantification from force-indentation plots
Models such as the Hertz model for spherical indenters can be used to extract quantitative measurements of cell elasticity and stiffness from the force-indentation curves.
See other video examples:
Video Cell indentation of a yeast cell. The data plot shows the force-indentation curves of several indentation steps.
Related applications:
Stretching of a yeast cell
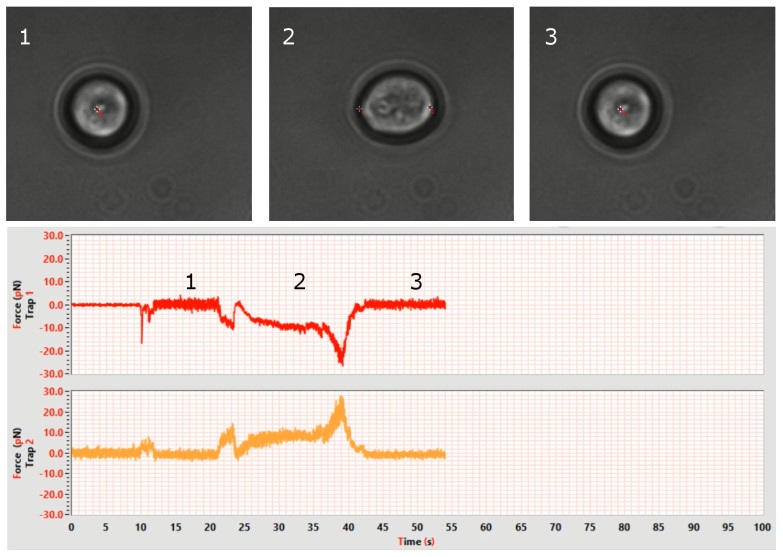
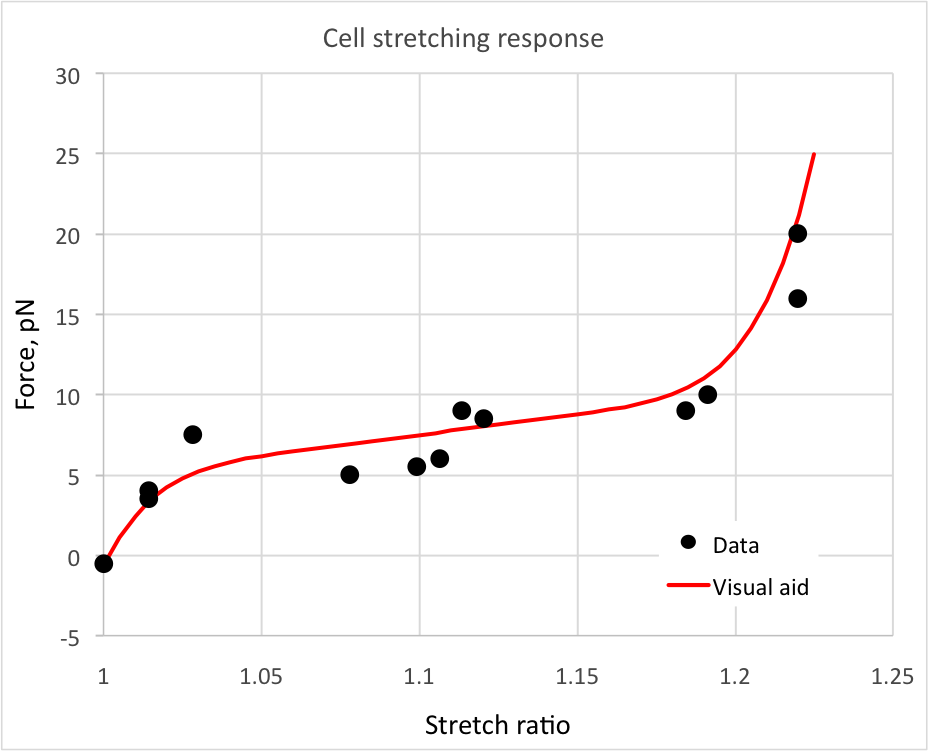
Fig. 2 Applied force vs stretch ratio for a yeast cell stretching experiment.
Key Concepts
-
- Cell rigidity or stiffness: refers to the ability of a material to resist deformation when subjected to an applied force.refers to the stiffness of the cell and how resistant it is to deformation.
- Cell elasticity: describes how the cell deforms and then returns to its original shape after the force is removed.
- Young’s Modulus: also known as Elastic Modulus, it is a quantitative measure of stiffness. A higher Young’s modulus indicates that a material is stiffer and less prone to deformation under stress. Matematically, it is defined as E=ϵ/σ where is the stress, defined as the force applied per unit area and is the strain, defined as the deformation per unit length.
- Indentation depth: the depth to which the bead indents the cell provides information about the mechanical resistance of the cell membrane and the underlying cellular structure.
Advantages
-
- Precision: optical tweezers allow for very precise control of the force and displacement, leading to accurate measurements.
- Quantitative data: the ability to measure forces in the picoNewton range and displacements in the nanometer range enables quantitative analysis of cell rigidity and elasticity.
- Non-invasive: optical tweezers are minimally invasive, reducing potential damage to the cells being studied.
- Localized measurements: the technique can target specific regions of the cell, providing localized information about mechanical properties.
Conclusions
Cell rigidity and elasticity measurements can be conducted in a simple and straightforward manner with SENSOCELL optical tweezers thanks to its calibration-free force sensor and extensive optical trapping features.
Would you like a DEMO?
Download SENSOCELL brochure